- Misconceptions
- Classroom Physics

S.H.M. with a cantilever

Class practical
This experiment could extend (or replace) the traditional pendulum or mass-on-a-spring experiments illustrating S.H.M.
Apparatus and Materials
- Metre rules
- Slotted masses ( 100 g each)
- Stopwatches
- Small, rough wooden blocks
Health & Safety and Technical Notes
It might be best advised to wear goggles in case something snaps.
Don't stand with toes underneath the slotted masses.
Read our standard health & safety guidance
- G-clamp the metre rule securely to the bench using the wooden blocks to protect the rule and bench.
- Sellotape one or more slotted masses near the end of the rule.
- Twang and time several oscillations.
- Adjust the vibrating length (or mass attached) and repeat.
Teaching Notes
- You could use this experiment as a follow-up to the standard "g from a pendulum". There are more variables to play with so you can easily set up differentiated tasks for your students.
- In this case we can find E for wood because for this cantilever we have ...
- x = width of ruler
- y = thickness of ruler (scale to undersurface)
- M = mass Sellotaped on
- L = vibrating length
- E = Young modulus of wood
- So T 2 v M (or L 3 ) gives you E from the gradient.
- With a wide range of abilities you can have one group simply verifying it's S.H.M. (by proving - T is independent of amplitude), another determining E , and another using log graphs to discover that T is proportional to - L 3/2 . It's also a good one for error analysis; which term contributes the largest error in E (answers on a postcard)?
- The vibrations are quite fast (especially at short lengths). To obtain an accurate result for T , time many oscillations and find the average time for a single oscillation.
- If you have the materials you can try things other than metre rules.
Thank you to Wayne Morton for pointing out that there was an error in the formula that we previously printed.
This experiment was submitted by Jason Welch who is Head of Physics at County High School, Leftwich, Cheshire.
Appears in these Collections
Simple harmonic motion.
Oscillations with a particular pattern of speeds and accelerations occur commonly in...
For 14-16 15 Resources
Supporting A-level practical requirements
There are two components to the assessment of practical work: Questions in the...
For 16-19 56 Resources
Other resources on Simple Harmonic Motion
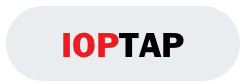
Episode 305: Energy in simple harmonic motion
Qualitatively, students will appreciate that there is a continuous change in the ways that energy is stored during simple...
Lesson 16-19
Oscillations, waves and mathematical models
Every mechanical wave has its origin in an oscillating object. The particles in the medium themselves oscillate, in a similar...
Teaching Guidance 14-16
Lath with a load
Demonstration: A demonstration experiment that makes a time trace of the oscillation and illustrates damping.
Practical Activity 14-16
Simple Harmonic Motion
Support our manifesto for change.
The IOP wants to support young people to fulfil their potential by doing physics. Please sign the manifesto today so that we can show our politicians there is widespread support for improving equity and inclusion across the education sector.
Physics Links Explorer
Stack Exchange Network
Stack Exchange network consists of 183 Q&A communities including Stack Overflow , the largest, most trusted online community for developers to learn, share their knowledge, and build their careers.
Q&A for work
Connect and share knowledge within a single location that is structured and easy to search.
Derive equation for a cantilever in SHM
I am currently investigating how a hacksaw blade's time period of oscillation changes when I add mass to the end of it or when I change the length it is clamped at.
I found the following equation from an IB worksheet:
$$ T^2=\frac{16M\pi^2x^3}{bEd^3} $$
- $T$ is the time period for one oscillation;
- $M$ is the mass of the cantilever;
- $x$ is the length of the cantilever;
- $b$ is the breadth of the cantilever and $d$ is the thickness of the cantilever;
- and $E$ is the stiffness of the cantilever.
I've looked around the internet and asked my teachers, but I haven't been able to derive this equation from first principles or the equations given in my syllabus.
If anyone knows where this comes from or would like to try and work it out, I would be very greatful.
- newtonian-mechanics
- harmonic-oscillator
- continuum-mechanics

- $\begingroup$ Did you look up beam vibrations? $\endgroup$ – John Alexiou Commented Mar 19, 2015 at 18:16
- $\begingroup$ Can you provide a sketch that indicates the supports and the direction of vibration (side to side, or up and down). $\endgroup$ – John Alexiou Commented Mar 19, 2015 at 18:18
- $\begingroup$ The cantilever's direction of motion is perpendicular to that of gravity, with the thin, long edge facing towards the ground. $\endgroup$ – DanielDC88 Commented Mar 22, 2015 at 20:27
- $\begingroup$ Can you please show some kind of sketch. $\endgroup$ – John Alexiou Commented Mar 23, 2015 at 3:13
- $\begingroup$ Sketch and Source Document $\endgroup$ – DanielDC88 Commented Mar 24, 2015 at 20:17
2 Answers 2
I was able to derive a similar equation, but not the exact one you mention.
The differential equation of a beam can be stated as
$$ E I \frac{\partial^4 u(z,t)}{\partial z^4}+\rho A \frac{\partial^2 u(z,t)}{\partial t^2} =0$$
where: $E$ is the elastic modulus, $I$ is the section area moment, $\rho$ is the mass density, $A$ is the section area and $u(z,t)$ is the deformation as a function of position $z$ and time $t$.
In your case, $$\begin{aligned} \rho A & = \frac{M}{x} \\ I & = \frac{1}{12} b d^3 \end{aligned}$$
To fit a static wave of the form $u(z,t)=\sin(\omega t)\sin(n\pi \frac{z}{x})$ you must have $$ \left(\frac{\pi^4 E b d^3 n^4}{12 x^4}-\frac{M \omega^2}{x} \right) u(z,t) =0$$ which is solved for $\omega = \frac{2\pi}{T}$ as
$$ T^2 = \frac{48 M x^3}{\pi^2 E b d^3 n^4} $$ with the first fundamental frequency when $n=1$. The above is for a simply supported beam, which fits the general shape of the wave $u(z,t)$. For other types of end conditions different wave shapes are needed and different frequencies arise.

- $\begingroup$ From the sketch I see now this is cantilever beam, and hence the above solution stands for $n=\frac{1}{2}$ or $$ T^2 = \frac{768 M x^3}{\pi^2 b E d^3} $$ $\endgroup$ – John Alexiou Commented Mar 24, 2015 at 21:22
The derivation is given in Module 10: Free Vibration of an Undampened 1D Cantilever Beam used by University of Connecticut School of Engineering. The derivation follows the same lines as given in ja74's answer, differing in the boundary conditions (top of p 3).
Note that the formula 10.7 $\gamma_n=\beta_n L=\frac12(2n-1)\pi$ in the UoC document appears to be incorrect, and in equation 10.10 $\beta_n$ should be $\beta_nL=\gamma_n$.

The lowest mode $(n=1, \gamma_1=1.875)$ applies in your case. From the dimensions of the beam we have $A=bd$ and $I=\frac{1}{12}bd^3$. Making these substitutions we get $$T_n=\frac{1}{f_n}=6.19\frac{L^2}{d}\sqrt{\frac{\rho }{E}}$$
In your equation we should substitute $M=\rho bdL$ and $x=L$. Then you have $$ T=4\pi \frac{L^2}{d}\sqrt{\frac{\rho }{E}}$$ which has the same form but is a factor of 2.03 larger.
Both equations agree that the relation is $T \propto \frac{L^2}{d}$. The main reason for the discrepancy in your equation is that an extra factor of $x=L$ is hidden within $M=\rho LA$.

Your Answer
Sign up or log in, post as a guest.
Required, but never shown
By clicking “Post Your Answer”, you agree to our terms of service and acknowledge you have read our privacy policy .
Not the answer you're looking for? Browse other questions tagged newtonian-mechanics harmonic-oscillator continuum-mechanics or ask your own question .
- Featured on Meta
- Preventing unauthorized automated access to the network
- User activation: Learnings and opportunities
- Join Stack Overflow’s CEO and me for the first Stack IRL Community Event in...
Hot Network Questions
- Is a private third party allowed to take things to court?
- Does copying files from one drive to another also copy previously deleted data from the drive one?
- Is the Earth still capable of massive volcanism, like the kind that caused the formation of the Siberian Traps?
- FIFO capture using cat not working as intended?
- How many jet fighters is Azerbaijan purchasing?
- Is “No Time To Die” the first Bond film to feature children?
- Why does the Rdson limit from a MOSFET’s SOA graph not match its output characteristics curve?
- Do early termination fees hold up in court?
- Why do evacuations result in so many injuries?
- Harmonizing modes other than major and minor
- Purpose of sleeve on sledge hammer handle
- Extracting linework from a GeoDataFrame
- Which Lebanese politicians, in the 2023-24 violence cycle, had called upon Hezbollah not to persist in attacking Israel from Lebanese soil?
- How old was Abraham when Rebecca (Isaac's wife) was born?
- Waiting girl's face
- Help. It's not compiling!
- How do I avoid getting depressed after receiving edits?
- How similar were the MC6800 and MOS 6502?
- Undamaged tire repeatedly deflating
- Player sprite becomes smaller during attack animation (Java)
- Were Soviet doctors and nurses actually as callous as "Voices from Chernobyl" portrays in the prologue?
- Are logic and mathematics the only fields in which certainty (proof) can be obtained?
- Story from the mid-20th century about a biochemist who finds youth algae
- Is there a name for this Fibonacci formula that uses hyperbolic trigonometry?
Search form

- Strength of Materials Lab
- Vibration Lab
- Simulation Labs
- Virtual Tour
- Video Tutorials
- Photo Gallery
Free vibration of cantilever beam
- Self Evaluation
- Remote Trigger
- Assignments
Free Vibration of Cantilever Beam - Theory
Learning Objectives
After completing this remote triggered experiment on free vibration of a cantilever beam one should be able to:
- Model a given real system to an equivalent simplified model of a cantilever beam with suitable assumptions / idealizations.
- Calculate the logarithmic decrement, damping ratio, damping frequency and natural frequency of the system
- Find the stiffness and the critical damping of the system.
- Calculate damping coefficient of the system.
Introduction
A system is said to be a cantilever beam system if one end of the system is rigidly fixed to a support and the other end is free to move. Look at few of the real systems shown below, try to make suitable assumptions to deduce the system to a cantilever beam.
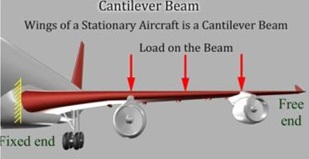
An aircraft wing as a cantilever beam An atomic force microscope probe
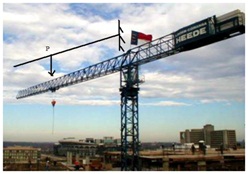
A tower crane overhang is like a cantilever beam A double overhang folding bridge
Vibration analysis of a cantilever beam system is important as it can explain and help us analyse a number of real life systems. As shown in above examples, real systems can be simplified to a cantilever beam, thereby helping us make design changes accordingly for the most efficient systems.
Natural Frequency of Cantilever Beam
When given an excitation and left to vibrate on its own, the frequency at which a cantilever beam will oscillate is its natural frequency. This condition is called Free vibration. The value of natural frequency depends only on system parameters of mass and stiffness. When a real system is approximated to a simple cantilever beam, some assumptions are made for modelling and analysis (Important assumptions for undamped system are given below):
- The mass ($m$) of the whole system is considered to be lumped at the free end of the beam
- No energy consuming element (damping) is present in the system i.e. undamped vibration
- The complex cross section and type of material of the real system has been simplified to equate to a Cantilever beam
The governing equation for such a system (spring mass system without damping under free vibration) is as below: $$ m\ddot{x}+kx= 0 $$$$ \ddot{x}+ω_n^2 x=0 $$ $$\omega_n = \sqrt{\frac k m} $$ $k$ , the stiffness of the system is a property which depends on the length ($l$), moment of inertia ($I$) and Young's Modulus ($E$) of the material of the beam and for a cantilever beam is given by: $$k=\frac{3EI}{l^3}$$
Damping in a Cantilever Beam
Although there is no visible damper (dashpot) the real system has some amount of damping present in it. When a system with damping undergoes free vibration the damping property must also be considered for the modelling and analysis.
Single degree of freedom mass spring damper system under free vibration is governed by the following differential equation: $$ m\ddot{x}+c\dot{x}+kx= 0 $$ $$ \ddot{x}+2\zeta\omega_n\dot{x}+\omega_n^2 x=0 $$ $c$ is the damping present in the system and $\zeta$ is the damping factor of the system which is nothing but ratio of damping $c$ and critical damping $c_c$. Critical damping can be seen as the damping just sufficient to avoid oscillations. At critical condition $\zeta =1$. For real systems the value of $\zeta$ is less than 1. For system where $ \zeta < 1 $ the differential equation solution is a pair of complex conjugates. The displacement solution is given by $$ x(t) = e^{- \zeta \omega_n t} \left[ x_0 cos (\omega_d t) + \frac {v_0 + \zeta \omega_n x_0} {\omega_d} sin (\omega_d t) \right] $$ where $x_0$ and $v_0$ are initial displacement and velocity and $\omega_d$ is the damped natural frequency of the system. The damped natural frequency is calculated as below: $$ \omega_d = \omega_n \sqrt {1- \zeta ^2} $$
Free Vibration of Cantilever Beam - Procedure
- Determine the damped natural frequency, logarithmic decrement and damping ratio of a given system from the free vibration response
- Calculate the mass of the system actively participating in dynamics
- Determine the equivalent viscous damping present in the system
- Calculate critical damping of the system and undamped natural frequency of the system
GENERAL INSTRUCTIONS
WE INSIST THAT USER READ THE INSTRUCTIONS & PROCEDURE THOROUGHLY BEFORE CARRYING OUT THE EXPERIMENT.
ONLINE and OFFLINE modes :
For the user to conduct an experiment, he/she must be connected to the SOLVE lab servers at NITK Surathkal . For this the user must be in the ONLINE mode. The user will be allowed to save the results of the online experiments and use the same for further analysis in OFFLINE mode. In case of OFFLINE mode the user is not connected to the experiment. However he/she can load a previously saved data file to get required graphs and carry out further analysis of the data. For this to happen, the user MUST have a Saved Data of the same experiment. Refer to step 6 below in procedure for downloading data.
CONTROL and VIEW modes :
In ONLINE mode, depending on availability of experiment, a user may get CONTROL or VIEW mode. In CONTROL mode the user gets to control the experiment parameters and trigger the experiment. In VIEW mode the user will not be able to control the experiment or trigger it, but will receive the data acquired during the experiment. The graph will load on screen once the data acquisition is complete. The VIEW user can chose to continue to calculations with the data received. ONLY ONE user is allowed to be in the CONTROL mode at any instant.
Allowed Actions during Remote Trigger :
While doing the experiment, i.e. when user is in Remote Trigger window, right click is permitted only in the graph area where the context menu will give options to zoom in, zoom out, save as image etc. Also, user MUST NOT REFRESH the page while carrying out an experiment. Page refreshing leads to loss of connection with the experiment server. However, the users may move back and forth between PROCEDURE tab and REMOTE TRIGGER tab while doing calculations, if they need to refer to formula given in table below.
Note: Time remaining and Number of Trials left are shown in INDICATOR block. If time is up or the number of trials left is zero the user automatically gets disconnected from the experiment server.
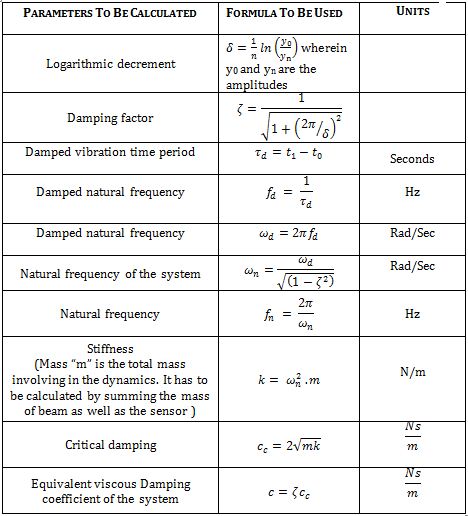
Effective mass of cantilever beam concentrated at the free end is 0.23m b eam . So for this experiment m= m sensor +0.23m beam
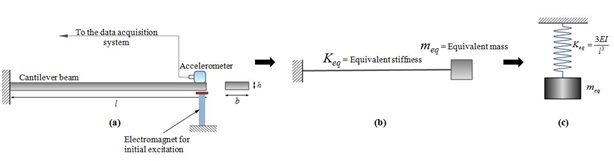
Fig 1: (a) Schematic of the experimental setup; (b) Equivalent engineering model; (c) Lumped parameter system
1. To conduct experiment click Start . In order to load previously saved data click on Choose file and locate the appropriate file. A pop-up dialogue box will inform you on successful loading of data. You can access the graphs and proceed to calculations once you click OK on the pop-up box. (Go to step 4 for calculations)
2. Once you are connected to the experiment server in CONTROL mode you can carry out the experiment. Depending upon the availability you will be granted CONTROL or VIEW mode. If you are granted VIEW mode you will be put in Queue and will be shown the waiting time for getting a CONTROL slot. You can either wait or you can chose to use the data acquired during ongoing session to proceed to calculations (Step 4)
3. Click on Trigger to actuate electromagnet. The electromagnet will attract and leave the free end of the cantilever beam due to which the cantilever beam will be set into free vibration. The acceleration data from accelerometer will load shortly on to your screen. Once you have received the data you may either choose to TRIGGER AGAIN or Disconnect from the experiment server. Number of trials left and the time left for you to finish the experiment are shown in Indicators block.
4. Click Proceed to Calculations to move to the calculations window. Known parameters are listed below the graphs. Hover over a point on the graph to find its x and y coordinates. Use available values to calculate listed parameters and enter them in appropriate fields. During calculations, the user may refer to table of formula given under the heading FORMULA above.
5. Click on Submit Calculations and View Results to submit your data. ONCE SUBMITTED ANSWERS CAN NOT BE REVERTED. A pop-up box will ask for your confirmation to submit the result. Click OK to CONTINUE. Click CANCEL if you wish to CHECK your data.
6. Click on Download Data button below the graph to download and save your data. You can use this file to load the graph and continue to calculations in the OFFLINE mode.
7. You may also save your results (for your record) by clicking Download Results. You will receive a CSV file containing the data of your results table.
Free Vibration of Cantilever Beam - Assignment
Try the following
- Length of Beam
- Lumped mass at free end of the beam
- What is the time taken to reduce vibration amplitude to half its initial value? How does it vary with damping ratio?
- Mechanical Vibrations by " Singiresu S. Rao ", Addison-Wesley Longman Incorporated, 1990
- Theory of Vibrations with Applications by " Chandramouli Padmanabhan , Marie Dillon Dahleh , William T. Thomson", Pearson Education, 2008
- Mechanical Vibration Practice and Noise Control by "V. Ramamurthi ", Narosa Publishing House, 2012
- Mechanical Vibration by " Haym Benaroya and Mark L. Nagurka ", CRC Press 2010
- Vibration and Acoustics by " Sujatha C", McGraw Hill Education, 2010
- Mechanical Vibrations by "G K Grover", NEM Chand & Bros, 2009
Related links
- Virtual Tour - NITK
Get in touch with us
Center for system design ( csd ).
NITK , Surathkal . P. O. Srinivasnagar , Mangalore - 575025, Karnataka , India
Tel: +91-824-2473915 Email: csd@ nitk .edu.in
You are here
Customized by CSD . Designed by WeebPal Drupal Themes . Powered by Drupal .
Sorry, your PHP version is not supported
Your host needs to use PHP version 8.1.0 or newer to run this version of Joomla!
Help me resolve this
- General Engineering
- Mechanical Engineering
- Electrical Engineering
- Aerospace Engineering
- Nuclear Engineering
- Materials Engineering
Follow along with the video below to see how to install our site as a web app on your home screen.
Note: This feature may not be available in some browsers.
- Engineering
Solved: Cantilever Experiment: Deriving Formulae
- Thread starter ash1098
- Start date Dec 14, 2007
- Tags Cantilever Experiment
- Dec 14, 2007
Attachments
- h.pdf 40.1 KB · Views: 2,938
- T.pdf 41.1 KB · Views: 2,069
- Unique straining affects phase transformations in silicon, a material vital for electronics
- Shark intestines inspire a new way to keep fluid flowing in one direction down a pipe
- New adhesive using elastomer makes lighter, more carbon-efficient vehicles possible
- Dec 21, 2007
Have you studied how to calculate bending moments and shear stresses in beams? That's the starting point. You will finding it covered in any standard Engineering Mechanics/Solid Mechanics/Strength of Materials textbook (e.g., Timoshenko) or online by Googling the above terms (also try deflection of beams). If you don't want to start from first principles, you can start with the general deflection equation for a cantilevered beam: [tex]h=\frac{PL^3}{3EI}[/tex] which is essentially, the cross-section independent form of your second equation. The equation for the time period of small oscillations comes from plugging the shear stress into the restoring force and linearizing to first order. Or if you'll settle for a quick wave of the hands, notice that the equation for T is exactly what you would get if you take the expression for h and plug this into the place of the length of a simple pendulum.
- Mar 19, 2008

FAQ: Solved: Cantilever Experiment: Deriving Formulae
1. what is a cantilever experiment.
A cantilever experiment is a scientific experiment that involves studying the behavior of a cantilever, which is a rigid structural element that is supported at only one end. In this type of experiment, the cantilever is subjected to various forces and the resulting deflections are measured and analyzed.
2. What is the purpose of the cantilever experiment?
The purpose of the cantilever experiment is to derive formulae that can be used to predict the behavior of cantilever structures under different loading conditions. These formulae can then be applied in engineering and design to ensure the structural integrity and stability of cantilevered structures.
3. What factors affect the behavior of a cantilever?
The behavior of a cantilever can be affected by several factors, including the material properties of the cantilever, the type and magnitude of applied load, the length and thickness of the cantilever, and the support conditions at the fixed end.
4. How are formulae derived from the cantilever experiment?
In a cantilever experiment, the deflection of the cantilever is measured for different applied loads. By analyzing these data, researchers can use principles of mechanics and mathematics to derive formulae that describe the relationship between the applied load and the resulting deflection of the cantilever.
5. How are the formulae from the cantilever experiment used in real-world applications?
The formulae derived from the cantilever experiment are used in engineering and design to ensure the structural safety and stability of cantilevered structures, such as bridges, balconies, and beams. They are also used in material testing and research to study the properties of different materials and their behavior under different loading conditions.
Similar threads
- Dec 15, 2007
- May 4, 2009
- Jul 20, 2008
- Jul 13, 2008
- Apr 6, 2010
- Nov 11, 2022
- Feb 26, 2020
- Jul 3, 2022
- Jul 31, 2015
- Feb 17, 2021
Hot Threads
- Wing Sails on modern ships
- How to stop/reduce ultrasonic sound wave device?
- How to attach clamp to metal bar?
- Extruding a curved tube of pasta dough
- Need help with determining thickness of steel bars
Recent Insights
- Insights Brownian Motions and Quantifying Randomness in Physical Systems
- Insights PBS Video Comment: “What If Physics IS NOT Describing Reality”
- Insights Aspects Behind the Concept of Dimension in Various Fields
- Insights Views On Complex Numbers
- Insights Addition of Velocities (Velocity Composition) in Special Relativity
- Insights Schrödinger’s Cat and the Qbit
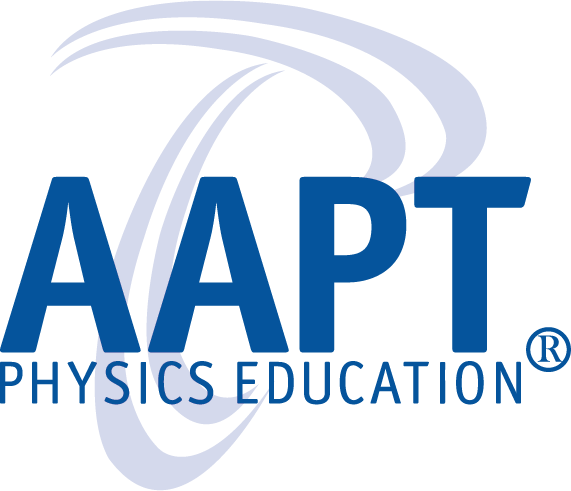
- Previous Article
- Next Article
An undergraduate experiment on the vibration of a cantilever and its application to the determination of Young’s modulus
- Article contents
- Figures & tables
- Supplementary Data
- Peer Review
- Reprints and Permissions
- Cite Icon Cite
- Search Site
Keith Turvey; An undergraduate experiment on the vibration of a cantilever and its application to the determination of Young’s modulus. Am. J. Phys. 1 May 1990; 58 (5): 483–487. https://doi.org/10.1119/1.16480
Download citation file:
- Ris (Zotero)
- Reference Manager
An experiment is described to test the theory for the frequencies of lateral vibrations of a uniform cantilever. Good agreement between experiment and theory is obtained. Young’s modulus for the cantilever material (steel) is determined from the observed frequencies of the fundamental mode for a series of different lengths, and the result is compared with that obtained from static deflection of the cantilever. The agreement between the dynamic and static determinations is satisfactory.
Citing articles via
Submit your article.
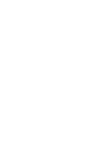
Sign up for alerts

Related Content
- Online ISSN 1943-2909
- Print ISSN 0002-9505
- For Researchers
- For Librarians
- For Advertisers
- Our Publishing Partners
- Physics Today
- Conference Proceedings
- Special Topics
pubs.aip.org
- Privacy Policy
- Terms of Use
Connect with AIP Publishing
This feature is available to subscribers only.
Sign In or Create an Account

- Theoretical background of SPM
- 2. Scanning Force Microscopy (SFM)
2.3 Linear Oscillations of Cantilever
Study of a cantilever oscillation is a rather science – intensive problem. In many cases the general solution to the cantilever equation of motion can not be obtained in an analytical form. However, if cantilever deflections from the equilibrium position are small, oscillations of the system will be described by well known theories.
In this chapter we consider in detail problems of possible canti-lever linear oscillations modeling it as a spring pendulum. Oscillatory systems described by linear motion equation are called linear.
- 2.3.1 Natural oscillations
- 2.3.2 Oscillations in the presence of friction
- 2.3.3 Oscillations in the presence of external periodic driving force
- 2.3.4 Cantilever small oscillations in a force field
- 2.3.5 Approach-retraction curves
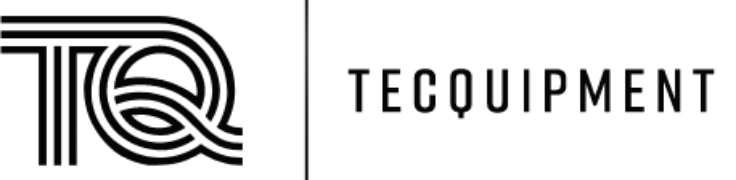
Product Ranges
Supporting products, global sales partners, select country/region to display contact and company details for your local tecquipment representative., applications.
- Case Studies
Find out how TecQuipment provide educational tools for industries.
Tecquipment develops in-house software to support and further enhance the experimental capabilities of our engineering lab equipment., highly commended businesses, tecquipment recognised by the department for business and trade (dbt) as a highly commended businesses in the inaugural made in the uk, sold to the world awards for the education category..
- Work with Us
- Aerodynamics
- Alternative Energy
- Control Engineering
- Electrical Power Systems
- Engineering Science
- Environmental Control
- Fluid Mechanics
- Materials Testing & Properties
- Next Generation Structures
- Process Control
- Statics Fundamentals
- Theory Of Machines
- Thermodynamics
- Agriculture
- Chemical And Pharmaceutical
- Construction
- Food And Drink
- Oil And Gas
- Renewable Energy
- Student Resource Area
- Diversity and Inclusion
- Modern Day Slavery
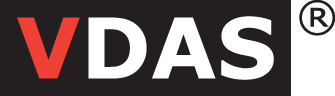
FREE VIBRATIONS OF A CANTILEVER
Experiment that uses the fundamental theory and Rayleigh's approximation to calculate the frequency of oscillation of a cantilever. Also utilises Dunkerley's method to predict the 'beam only' frequency. Fits on to the Free Vibrations Test Frame.
OR CALL US TO DISCUSS +44 1159 722 611
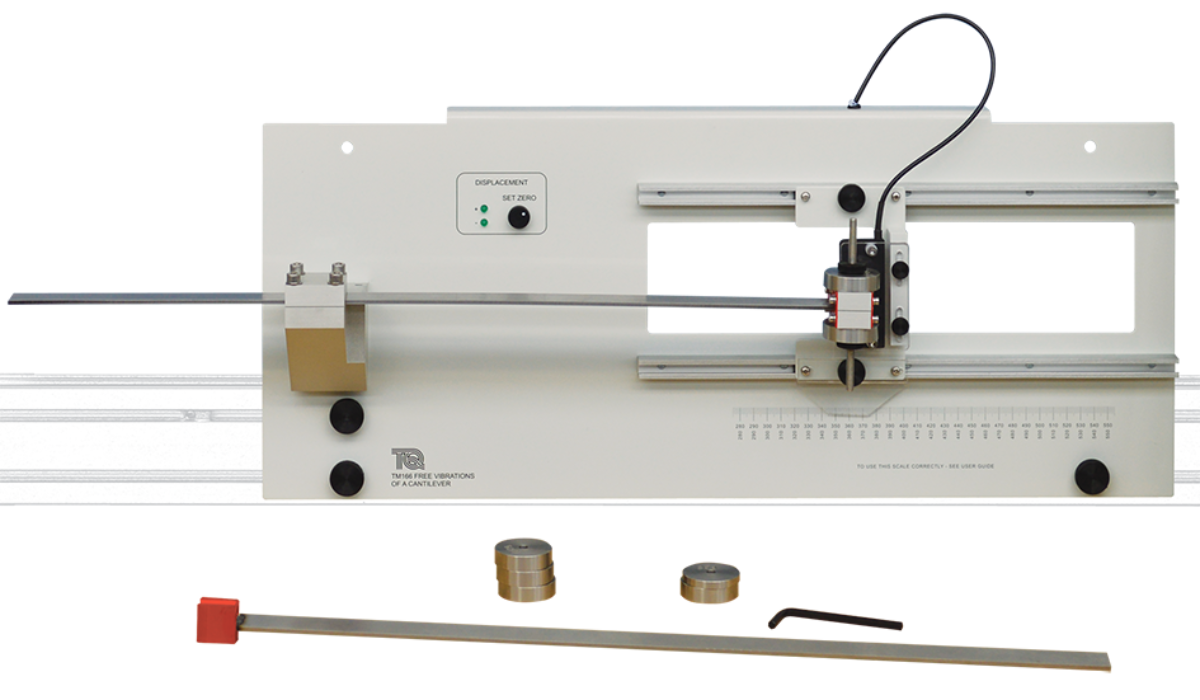
This product is part of a range that explores free vibrations in simple ‘one degree of freedom’ systems.
It introduces students to key scientific terms such as:
- Simple harmonic motion (SHM) and frequency of oscillation
- Beam stiffness
- Rayleigh’s method
- Dunkerley’s method
- Second moment of area
- Phase difference between displacement and its derivatives
This product fits to the sturdy Test Frame (TM160) for study or demonstration.
A beam with the mass at the end works in a similar way to a mass spring system - the stiffness of the beam simply replaces the stiffness of the spring. However, in a mass spring system, we normally assume a ‘light’ spring compared to the mass. The vibrating cantilever examines what happens if the spring element (the beam in this case) is not light. Additionally, it examines a beam (with no tip mass) as a complete self-contained system, forming the mass and the spring.
The vibrating cantilever forms a simple and highly visual example of oscillations that may occur in real structures such as aircraft wings.
A back panel fixes to the Test Frame. The panel holds a sturdy clamp and two runners. The clamp holds the beam. Students use the clamp to adjust the oscillating length of the cantilever. The runners hold a non-contacting sensor that measures the oscillations at the end of the cantilever. The sensor has no physical contact with the beam, for negligible damping.
The back panel has a printed scale. Students use it to set the beam length accurately.
The product includes two beams; a plain beam and a beam with tip mass. Students may add extra ‘tip mass’ to the second beam to test how it affects oscillations. Students pull the end of the cantilever down and release, allowing it to vibrate. They then find the frequency of oscillation and compare it with that predicted from theory. Students test the beam with a varying tip mass, changing the ratio of tip mass to beam mass. They discover that for most ratios, the assumption that the beam is ‘light’ may not give accurate predictions of oscillation frequency. They then learn how Rayleigh’s method improves the overall prediction. They also use Dunkerley’s method to predict the natural frequency of the beam only, comparing this value with that found by other methods.
The back panel fixes in both horizontal and vertical direction to allow students to test the beams in both positions.
TecQuipment calibrate the displacement sensor to work with VDAS® (mkII) for real-time display and data acquisition of system oscillation wave-forms. Students use the software to see the displacement waveform and measure frequency. The software calculates and shows the first two derivatives of displacement - velocity and acceleration.
TecQuipment have specifically designed the TM166 to work with VDAS® (mkII). However, the sensor output may be connected to your own data acquisition system or oscilloscope if desired.
View the Free Vibrations experiment flyer here.
Learning outcomes
- Predicting oscillation frequency using Rayleigh’s method and the simplified method assuming that the beam is ‘light’
- Horizontal cantilever length and frequency of oscillation
- Using Dunkerley’s method to predict the ‘beam only’ frequency
- Comparison of vertical and horizontal cantilevers
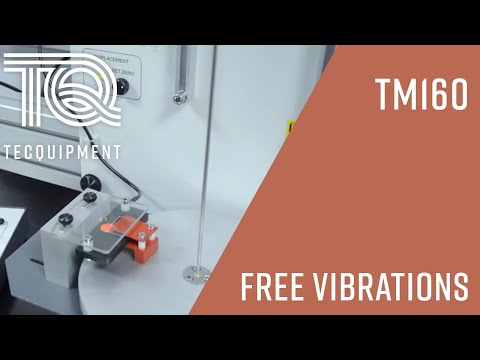
Free Vibrations TM160 Series - TecQuipment
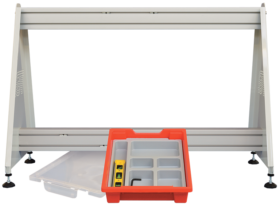
FREE VIBRATIONS TEST FRAME
A sturdy bench top frame for use with the Free Vibrations experiment modules.
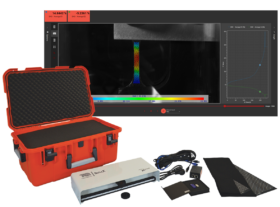
An entry-level digital image correlation (DIC) system to teach students how to measure and visualise surface deformations, strains and displacements in materials and shapes.
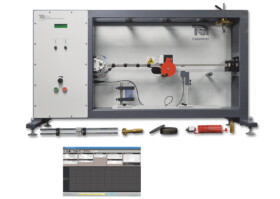
FREE AND FORCED VIBRATIONS

Bench top apparatus that investigates the free and forced vibrations of a rigid beam with a spring, and a simply supported beam. Demonstrates Rayleigh's approximation and Dunkerley's method.
NEED MORE INFORMATION?
We're here to answer your questions
SIGN-UP FOR THE LATEST INDUSTRY NEWS
Manufacturing the finest engineering education equipment for 60+ years.

IMAGES
VIDEO
COMMENTS
Fig. 4.1(a) shows of a cantilever beam with rectangular cross section, which can be subjected to bending vibration by giving a small initial displacement at the free end; and Fig. 4.1(b) depicts of cantilever beam under the free vibration. We have following boundary conditions for a cantilever beam (Fig. 4.1)
Twang and time several oscillations. Adjust the vibrating length (or mass attached) and repeat. Teaching Notes. You could use this experiment as a follow-up to the standard "g from a pendulum". There are more variables to play with so you can easily set up differentiated tasks for your students.
The following files can be downloaded to view an animation of the first few modes and total vibration of a cantilever beam. To view these, the Lotus Screencam Player must be downloaded: Scplayer.exe. Total motion: 325Htot.exe 1st mode: 325h1st.exe 2nd mode: 325h2nd.exe 3rd mode: 325h3rd.exe 4th mode: 325h4th.exe.
Build the photo-gate as described in figures 2-4. Details: Build the photo-gate as near to the end as possible so that the cantilever can extend beyond the bread-board and not hit it when vibrating. Make sure all of the wires are long enough to be pulled out of the way. Make sure to check the polarity of the LED.
The virtual experiment program of forced vibration of single DOF cantilever beam is divided in many sections. The step by step description of program is as follows which also include the guideline to proceed in the virtual experiment… 1. Title Page - This is the first page of virtual experiment. It includes title ...
cantilever beam with dimensions 1" width x 0.5" thickness x 32" length. The vibration occurs along the horizontal axis of the width. Equation 2 gives the partial differential equation that models this behavior. [2] In Equation 2, ü(x,t) is the deformation of the beam at location x and time t, the L
The derivation is given in Module 10: Free Vibration of an Undampened 1D Cantilever Beam used by University of Connecticut School of Engineering. The derivation follows the same lines as given in ja74's answer, differing in the boundary conditions (top of p 3). Note that the formula 10.7 $\gamma_n=\beta_n L=\frac12(2n-1)\pi$ in the UoC document appears to be incorrect, and in equation 10.10 ...
In this experiment, a small mass is connected at a distance to the rotor of a motor. This mass on the motor acts as an imbalance. When the motor is running, the cantilever beam experiences an external harmonic excitation. The experimental set up of the system is shown in Figure 8.
If the cantilever was released from varying initial points throughout the experiment, it would lead to major inconsistencies, as the total distance covered during the oscillation would be non-consistent, thereby affecting the total time of oscillation as well. The cantilever will be released 35 cm ± 0.05 cm lower than the initial
Experiment . Discussion . Quiz . Feedback . Webcam . 2.3 Theoretical natural frequency for cantilever beam . Fig. 2.1 (a): A cantilever beam . Fig. 2.1 (b): The beam under free vibration . A cantilever beam with rectangular cross-section is shown in Fig. 2.1(a). Bending vibration can be generated by giving an initial displacement at the free ...
Simple harmonic oscillations of cantilever and Find young's modulus of the bar-Anna university 2021 Regulation -physics laboratory
where ω 0 = k / m — frequency of natural undamped oscillations or the natural frequency. The cantilever eigenfrequency ω0 has been calculated in chapter 2.1.6. Solution of equation (1.1) with initial conditions z t = 0 = z 0 and dz dt t= 0 =v 0 is given by. z ( t ) = Z cos( ω 0 t + φ 0 ) , (1.2) 2. Z = z 2.
Natural Frequency of Cantilever Beam. When given an excitation and left to vibrate on its own, the frequency at which a cantilever beam will oscillate is its natural frequency. This condition is called Free vibration. The value of natural frequency depends only on system parameters of mass and stiffness. When a real system is approximated to a ...
Procedure: 1. Use the apparatus, set up as shown in the diagram. Start with a length of 0.900m (90.0cm). 2. Use a stop-watch to measure the time taken for 10 complete oscillations. However, if the total time is less than 10 seconds you should use more than 10 oscillations. Repeat twice more to obtain two more values. 3.
Solved: Cantilever Experiment: Deriving Formulae. ash1098. Dec 14, 2007. Cantilever Experiment. In summary, the two formulae for cantilever oscillations are T= 2 (pi)* [ (4ML^3)/ (bd^3E)]^1/2 and h= 4MgL^3/Ebd^3. The equation for the time period of small oscillations comes from plugging the shear stress into the restoring force and linearizing ...
An experiment is described to test the theory for the frequencies of lateral vibrations of a uniform cantilever. Good agreement between experiment and theory is obtained. Young's modulus for the cantilever material (steel) is determined from the observed frequencies of the fundamental mode for a series of different lengths, and the result is ...
Bending, Buckling, and Vibration David M. Parks 2.002 Mechanics and Materials II Department of Mechanical Engineering MIT February 9, 2004. Linear Elastic Beam Theory ... -Constitutive equations •Applications: -Cantilever beam deflection -Buckling of beams under axial compression -Vibration of beams. Beam Theory: Slice Equilibrium ...
Candilever - Youngs ModulusOn screen Soumya M ( Assistant professor of physics Dept 2020)
In this chapter we consider in detail problems of possible canti-lever linear oscillations modeling it as a spring pendulum. Oscillatory systems described by linear motion equation are called linear. 2.3.1 Natural oscillations. 2.3.2 Oscillations in the presence of friction. 2.3.3 Oscillations in the presence of external periodic driving force.
Is the frequency of a damped free vibration smaller or greater than the natural frequency of a system? Why? What is the basic difference between the free responses exhibited by an under damped system and an over damped system? A damped free oscillation amplitude is observed to be reduced to 20% of its initial amplitude in 100 complete cycles.
The cantilever does not respond instantly to perturbations in oscillation amplitude. The cantilever drive system pumps energy gradually into the cantilever oscillation. To demonstrate the conflicting requirements, figure 1 illustrates a typical response curve of the cantilever amplitude as a function of time, with the performance of the system ...
Experiment that uses the fundamental theory and Rayleigh's approximation to calculate the frequency of oscillation of a cantilever. Also utilises Dunkerley's method to predict the 'beam only' frequency. ... The runners hold a non-contacting sensor that measures the oscillations at the end of the cantilever. The sensor has no physical contact ...
Download: Download high-res image (404KB) Download: Download full-size image Fig. 3. Different steps of the image processing algorithm: (a) importing one frame of a video footage; (b) detecting the edges of the cantilever and the clamped base in the frame; (c) removing the clamped base edges and resetting the position so that the base is at the coordinate origin; (d) converting the edge ...